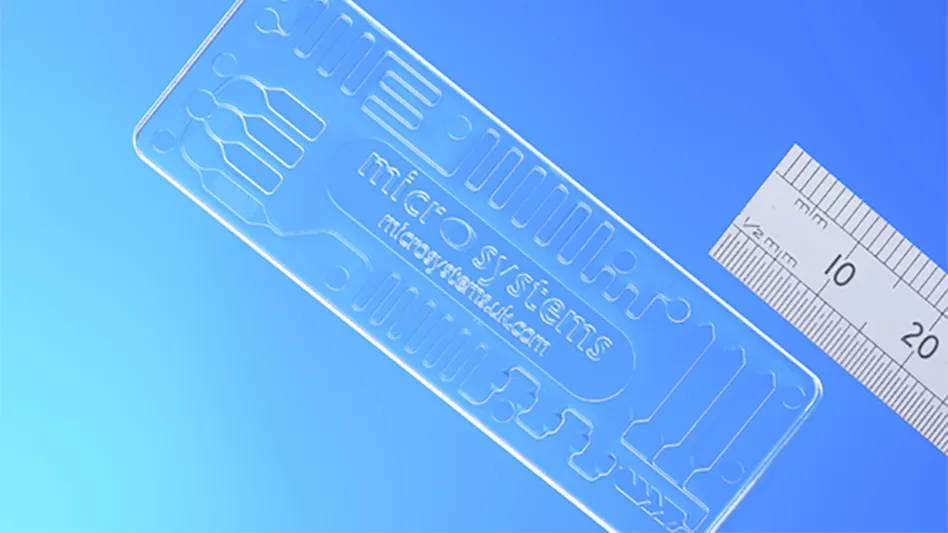
Micro Systems
With the latest developments in the field of biotechnology, microfluidic devices have shown its enormous potential for commercial success. Pharmaceuticals, drug discovery, point-of-care (POC) diagnostics, next-generation sequencing, cell analysis, biomarker research, and other fields are just some examples of the potential fields of microfluidic technology. According to a recent report, by 2032, the microfluidics market is expected to be valued at US$81.36 billion, indicating that it is poised for substantial growth with a notable compound annual growth rate of 15.1% between 2023 and 2032.
Moving from prototypes to mass production of microfluidics devices
The differences between academia and industry present several difficulties throughout the translation process, particularly when moving from laboratory prototype to industrial scale-up production, which is essential for possible commercialization of new microfluidics devices. Beside challenges in research funding, closing the gap between end users and technologies, and other exhaustive procedures like patent licensing and regulatory approval, a successful commercialization of microfluidics devices requires thought from the initial design of prototype stages to ensure the product can be effectively scaled up for mass production.
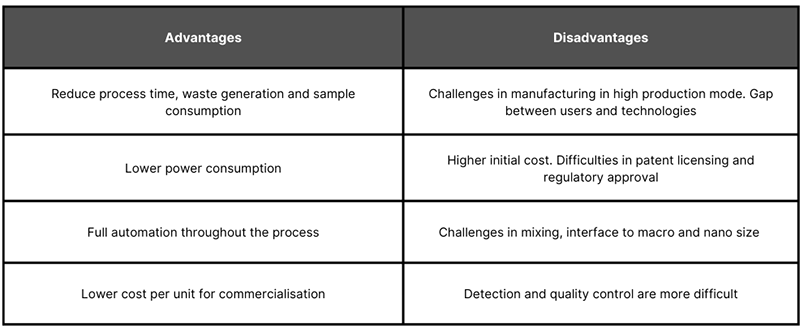
An initial challenge lies in the transition from prototype molds (1 to 2 cavities) to multi-cavity mass-production molds, as the heavily invested mass-production molds need to uphold the highest requirements in precision and accuracy in repeatedly reproducing microfluidic devices with dimension down to nano levels. In practice, different manufacturing methods are utilized at different development stages of microfluidics and nanofluidics, starting from silicon or glass (low scale traditional microelectronics), to elastomers (medium scale production), to thermoplastic and lamination (mass production).
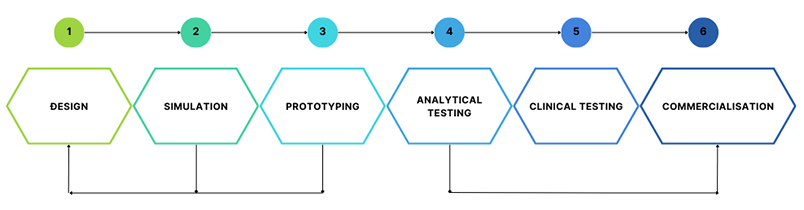
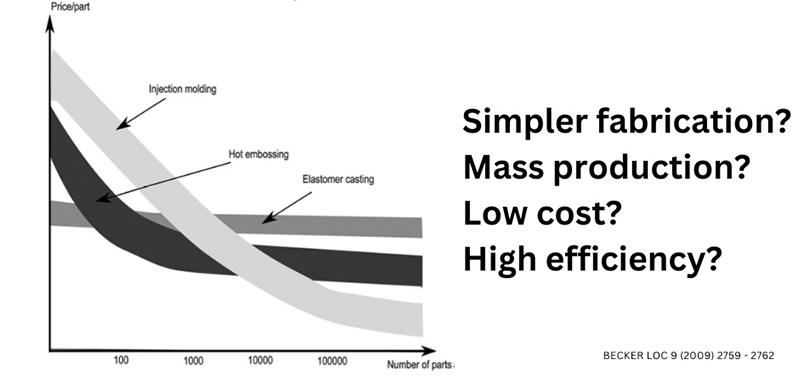
Generally, different techniques can be applied for the replication of plastic microfluidics parts, including imprint/embossing, injection molding and casting. For a successful mass production to tens of thousands and millions of microfluidics parts, microfluidic molds need to have accurate patterning with the required feature size and aspect ratio, sufficient strength and durability, the capability to demold with a workable draught angle, a proper surface finishing and a favorable cost/performance ratio (Cong & Zhang, 2022). These micro ultra-precision molds are manufactured using advanced micro-machining (hardened steel) or micro-electroforming process (nickel plate) in a temperature-controlled environment with minimal vibration. For example, micro milling is a powerful method for producing extremely complex 3D geometries in challenging-to-manufacture materials like hardened steels for microfluidics molds. This method can be particularly useful for producing extremely accurate and precise parts with microscale features of less than 1-micron tolerance on the object's form and dimensional accuracy. The incredibly high speed (50,000+ rpm) lowers tool breakage, boosts productivity, and enhances cut and surface finish quality, making it possible to create precisely patterned surface microstructures and extremely accurate high-aspect ratio microstructures (microchannels, ultra-thin walls, and microarrays) for microfluidics molds.
For mass production, plastic injection molding, in this particular case, micro plastic injection molding, is a popular choice for manufacturers to effectively produce disposable medical applications like microfluidic devices, in a cost-effective and time-saving way with high automation level and repeatability. However, the complication in multiple materials (hence variations in shrinkage and tolerances) and complex manufacturing processes increase the risk of failures in microfluidics mass-production. Microfluidics devices of different materials might also require different manufacturing technologies, hence multi-material manufacturing methods and further assembly steps are necessary. In addition, as microfluidic devices feature mass production with their large aspect ratio structures and thin-walled geometries (Hoyle, R, 2007), thorough examination is required to ensure that the cavities are completely filled before the hardening layers start to obstruct the melt flow (Attia & Alcock, 2009). A number of factors within the micro injection molding process, including injection speed and pressure, mold temperature, holding time and clamping pressure, cooling time, can affect the reproducibility of microfluidics parts, especially when in combination with a variety of material properties and complex part geometries.
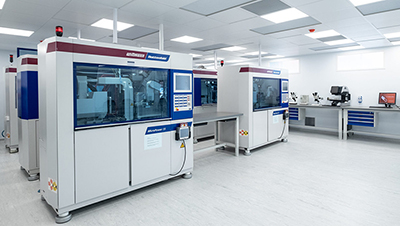
for replication of micro-featured microfluidic parts with
high accuracy. For medical purposes, microfluidics devices
are normally manufactured in ISO class cleanroom
environments.
In both mold making and micro injection molding processes to manufacture microfluidics parts, an exceptional micro metrology standard is necessary for precise and repeatable production. A variety of advanced micro metrology technologies and systems are needed for quality assurance of all micro parts manufactured on different length scales. For instance, non-contact white light interferometry is used for surface measurement and inspection, while focus-variation is used for shape and roughness measurement, producing color 3D models that can be compared to CAD models and used for other data analysis. Precisely scanning and measuring produced products as they are being created can help reduce project lead times, which can lead to faster design or steel iteration turnaround times, more accurate measurements, and possibly reduced prices.
For a successful mass production process, the appropriate manufacturing technology should be chosen depending on the required materials, process conditions and its end applications. It is hence extremely important for product designers and developers to build a strong relationship with experienced micro manufacturing providers early in the process, ideally a micro injection molder with in-house tooling capabilities, as a lot of time, the right experience and knowledge can save valuable time, resources and labor during the manufacturing process and mold process optimization in preparation for microfluidic chip manufacture on a mass scale.
Manufacturing professionals should also be involved in the early development stages, including the studying of the chosen material properties and physics on molding and demolding, as their extensive experiences can help with the feasibility of the project, reducing mass production failures and improving the cost-effectiveness of the product commercialization at later stages. Especially, with manufacturing professionals’ inputs and knowledge, designers can overcome future scale-up challenges by improving their design for manufacture (channels, ports, chambers, etc. or using a more commercialized material choice or adjusting aspects of the design to enable mass production), leading to significant savings in turnaround time and manufacturing cost (both tooling and replication).
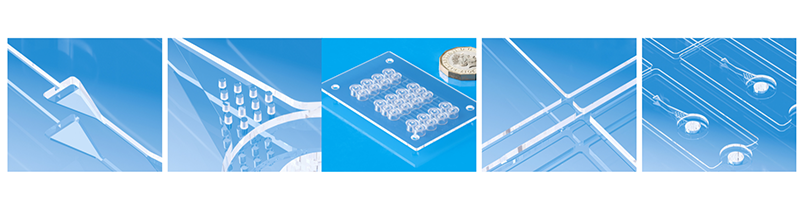
Microfluidics devices prove their great benefits in bringing efficient, portable, and less time-consuming solutions for a range of applications including point-of-care diagnostics and personalized medicine, at a reasonable cost. By developing the technology needed to regulate size and dimensions, repeatability, controlled surface qualities, low cost, etc., mass production manufacturers may overcome current hurdles. As the microfluidics device market is growing fast, the mass production of microfluidics devices is expected to receive more attention and investment, with promising innovative manufacturing solutions to come for microfluidic and nanofluidic devices fabrication.
Reference:
Cong, H. and Zhang, N. (2022) ‘Perspectives in translating microfluidic devices from laboratory prototyping into scale-up production’, Biomicrofluidics, 16(2). doi:10.1063/5.0079045.
Hoyle,R.Creatingmicroandnanostructuresinpolymersbymoulding.Med.DeviceTechnol.2007,18,18–20.
Attia,U.M.;Alcock,J.R.And Evaluation In Process-parameter part-geometry effects on the quality of filling in micro-injection moulding. Microsyst. Technol. 2009, 15,1861–1872.
Becker Lab on a Chip 9 (2009) 2759 - 2762)
Latest from Today's Medical Developments
- Lumetric's Scanning Tubing Measurement System
- Latest advancements in machine tool technology
- Visit Okuma America at IMTS 2024
- March 2024 USMTO orders were $354.7 million
- AM for Aircraft Cockpit Interior Components
- Arizona WearTech Center member receives new patent
- SABIC showcased progress in plastic innovation
- Ink for 3D-printing flexible devices, no mechanical joints